Feature: MSU's Superconducting Cyclotron Lab Unlocks Secrets of the Universe
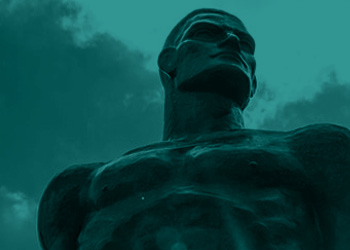
Nuclear science is devoted to answering nature’s most basic questions, and MSU aims to remain an international leader in this quest and also to educate nuclear scientists of the future.
THE CREATION
We’ve all heard about the Big Bang, but it only represents the beginning.
In a brick building by the Bogue Street roundabout, nuclear physicists at MSU’s National Superconducting Cyclotron Laboratory (NSCL) spend their days smashing atomic nuclei together to recreate the cosmic events that helped give birth to the heavy elements.
“Everything I am doing is to try to understand how we are here,” says Jorge Pereira, a nuclear astrophysics postdoctoral research associate. “When you look at a piece of lead, it represents one of the biggest questions. How was this lead created, and why in this amount? The truth is, we don’t know. And not only lead, but gold, silver—the elements that you know well.”
Elements are the fundamental materials that make up all matter in the universe and more than 90 of them exist. Though scientists know the processes that formed the 26 lighter elements on the Periodic Table inside stars, how the elements heavier than iron came to be remains uncertain. They know that many are created, even today, when large stars die and become cosmic explosions known as supernovae. But most of the specific nuclear reactions remain un-deciphered.
So researchers apply the modern tools of NSCL to probe the history of the cosmos. In doing so, they also hope to peer into the deepest secrets of the elements today.
THE CENTER
One champion of the cause is Rhiannon Meharchand, a second-year graduate student, who came from Florida to East Lansing to spend the next half decade knocking around the nuclear billiard balls, protons and neutrons, that make up nuclei, or the centers of atoms.
“I think it’s just the right size for me,” she says of nuclear physics, noting that other branches of physics examine even smaller particles than protons and neutrons, or look on a larger scale at atoms, molecules or heavenly bodies from afar. But more than just the nuclei, she says, “I like the size of the groups that work in nuclear physics, and I like the size of the equipment that you work with.”
Meharchand chose MSU because it had a “way better program” than her warmer-weather alternative. Namely, MSU boasts the second-highest ranked nuclear science graduate program in the country after M.I.T., according to the U.S. News & World Report in 2007. A National Science Foundation-funded institution, NSCL holds education as a high priority. About one-third of the 300 people that work at the lab are students, half of them undergraduates. Each year NSCL, the largest university-based nuclear science research facility in the country, delivers 10 percent of the nation’s nuclear science PhDs.
“Everyone is very willing to sit down and talk with you when you have questions,” notes Jill Pinter, a second-year graduate student. “That just shows how the NSCL stresses graduate education.”
Meharchand’s research consists of shooting beams of heavy nuclei traveling at nearly half the speed of light—fast enough to circle the Earth three times in one second—at a fixed target. As the nuclei collide they shatter and immediately recombine new nuclei. Of the thousands of types of reactions, Meharchand is looking for the rare reaction that knocks out one neutron and replaces it with a proton. It is like throwing a watermelon at a chocolate chip cookie and replacing one chocolate chip with a seed.
Examining what happens in this reaction will help model a process inside supernovae that is essential to the formation of new elements, a reaction where a neutron spontaneously becomes a proton. Since the number of protons in a nucleus defines an element, the phenomenon represents the final step in the birth of a new element.
At NSCL, a pair of cyclotrons speeds up nuclei and, like a laser, shoots them through a thin foil of the target material. Downstream from the target, a complex system of magnets filters out the desired nuclei. One out of ten million nuclei is the one that Meharchand wants.
“It’s like a very sophisticated microscope that looks at the nucleus of atoms,” says Brad Sherrill, a University Distinguished Professor, of the entire NSCL facility.
Beyond chasing cosmic explosions, however, scientists also want to know why nuclei are the way they are. Some nuclei, for example, will change shape from round to frisbee-like to football-shaped. “There are no models that can fully predict the properties of nuclei,” says Hendrik Schatz, a professor at NSCL. “We’ve just started scraping at the surface of understanding them.”
THE MACHINES
Walking east down the long hallway of NSCL’s office complex, you cross a line where the floor tiles change color and take on a faint rose hue. Here begins the original NSCL structure, erected in 1961 under the leadership of founding director Henry Blosser, now University Distinguished Professor Emeritus.
But the change is more than an aesthetic one. It is also one of trade. Around the corner is the engineers’ area, where Matt Johnson, a member of NSCL’s mechanical design team, nimbly manipulates a three-dimensional image of a tube-shaped gadget on his computer screen.
The apparatus, a radiofrequency cavity, is part of a next-generation linear accelerator that will allow ultra-fast beams from the cyclotron that are purposely stopped to be sped up again, but to lower speeds. The reaccelerator will allow physicists to cause previously inaccessible collisions that only work at lower speeds and examine more nuclear properties than ever before.
When Johnson left his hometown of Shepherd to attend a small college near Flint, he was planning on a career designing cars. When his program was cancelled due to low enrollment, he transferred to MSU, took up mechanical engineering, and landed a student job building magnets with NSCL. Upon graduating in 2001 he was immediately hired full time.
“It’s a lot more interesting than cars,” Johnson says. “One thing that’s nice about it here is that there’s always something new that hasn’t been done before. There’s always the fresh aspect of starting something from scratch, which is very nice.”
After his team finishes a three-dimensional digital blueprint, it will pass to the lab’s machine shop, where machinists use computer-guided tools to cut metal into custom shapes. To be assembled, the parts will make half a dozen trips to a special welder in Chicago and half a dozen more to NSCL’s clean room, according to Laura Saxton, a 2003 graduate in chemical engineering and clean room manager. Any piece of dust remaining during experiments, she says, would destroy the equipment in their ultra-high voltage environments.
BEYOND THE BEAM
A little farther down the building’s central byway, two dozen middle schoolers sit in a classroom gawking at Zach Constan, the lab’s outreach coordinator, who pours frothing, frigid liquid nitrogen on a quarter-sized disk.
More than 4,000 people toured NSCL last year under the guidance of Constan and a dozen graduate students. In addition to leading almost daily tours, during the summer he brings groups of middle- and high-school science teachers in for a week-long, free boot camp on nuclear physics.
“They are going to go back to their classrooms and reach far more students than we ever could,” he said.
But NSCL does not only display its equipment to visitors. Any scientist who wants to use the coupled cyclotron lab for research can apply. A national user facility, NSCL has a community of experimenters that includes more than 700 scientists from 35 countries.
Warren Rogers, professor of physics at Westmont College in Southern California, is a participant in a unique user-based collaboration. He belongs to a group of eight undergraduate institutions that bring students to NSCL every summer to work on MoNA, a device that detects neutrons that fly off of nuclear collisions.
“We don’t have these kinds of facilities at smaller colleges, so to be able to bring students here is a big plus,” he says.
Research at NSCL has gone beyond shaping theories about the physical world; it has also created instruments to improve it. From medical cyclotrons used in cancer radiation therapy to new tools for biotech and nanoscience research, NSCL accelerator technology has penetrated many fields. (See sidebar for more benefits of nuclear science.)
THE FUTURE
As many as 8,000 nuclei are predicted to exist, but so far only 2,000 have been observed, physicists say.
“In order to understand the interaction between neutrons and protons, the most important information is at the limits,” says Michael Thoennessen, professor and associate director for nuclear science.
The limits lie in rare isotopes, which are unstable nuclei with unique combinations of protons and neutrons that are too short-lived to be found on Earth. Extreme conditions in fast-beam collisions can produce them in tiny amounts sufficient to study their properties.
Expanding the limits requires a new generation of facilities, scientists say.
NSCL has proposed the development of a linear accelerator to replace the coupled cyclotron facility. The new machine would speed up particles in a straight line instead of around in a spiral. It would generate beams 100,000 times more powerful than the current facility’s—thus making it possible to probe much rarer exotic isotopes.
“I think there’s an overwhelming amount that we can study,” Pinter said. “That’s what excites me in nuclear science, this unknown.”
In 2006, NSCL, currently the top rare isotope research facility in the U.S., published a 400-page whitepaper detailing the scientific motivation and preliminary plans for the new facility.
The three other top rare isotope labs in the world—located in Japan, Germany, and France—are all undergoing major upgrades. One, RIKEN in Japan, began operating its new accelerator in December 2006.
“For us the issue is really the international competition,” says NSCL director Konrad Gelbke. If the upgrade does not go through, he says, international facilities will soon trump NSCL in capability. “An experiment that we would work on for an entire year, they could do in eight hours,” he says, “which means you can’t compete.”
Last fall the National Science Foundation awarded NSCL a $100 million grant to fund the lab until 2011. Support for the lab’s research culminated in a visit by NSF director Arden Bement and several members of Michigan’s congressional delegation—Sen. Carl Levin and Reps. Vernon Ehlers and Mike Rogers.
Jack Lessenberry, a prominent Michigan journalist and Detroit native, brings the issue home. He writes, “Having a first-class cyclotron laboratory is exactly the sort of thing we need to attract the sort of high-tech, new economy community Michigan needs.”
America’s scientific status may be undecided, but it is not the first time in history that understanding the stars has been intimately linked to the prospering of a society. The Ancient Greeks’ ability to sail by the skies helped to win them ancient glory and everlasting prestige.
With any luck, rare isotope research will bring us, too, to new shores.
Annie Jia spent the summer of 2007 writing about nuclear physics at NSCL. She has also written for the CERN Courier, symmetry magazine, the Stanford Daily, and the Stanford Report. She can be reached at anniejia@gmail.com. To schedule a tour of NSCL, email Zach Constan at constan@nscl.msu.edu.
THE MANY USES OF AMS
Nuclear science often yields applications outside of nuclear science. For example, it led to the invention of Accelerator Mass Spectrometry (AMS), a very sensitive way to measure radioactive isotopes—which has turned out to be extremely useful with a wide spectrum of benefits. Here are some AMS applications:
- Art: Irreplaceable artwork can now be analyzed without harm by taking less than one thousandth of a gram of pigment.
- Archaeology: Measuring the amount of carbon-14 in an artifact can yield its age.
- Climate: Geophysicists use AMS to track how long water in different parts of the ocean has been at the surface, allowing them to map currents that affect climate change.
- Nuclear waste: AMS can detect very low-level seepage around waste sites before it turns into a major problem.
- Radiation: AMS can detect iodine-129 in Chernobyl victims to determine their radiation exposure nine years before.
WERE YOU WONDERING?
- What is a cyclotron? A cyclotron is a machine that speeds up nuclei in a spiral to up to half the speed of light.
- What is a nucleus? A nucleus is the very center of an atom. It is 10,000 times smaller than the whole atom. If the atom were the size of a football field, the nucleus would be about as big as a marble.
- How fast do they go? The cyclotron shoots the nuclei out at half the speed of light—fast enough to orbit the Earth three times in one second!
- Is the cyclotron a nuclear reactor? No. Though nuclear energy is based on nuclear science, NSCL neither does research nor produce nuclear power.
- Is the cyclotron a robot? No. The cyclotron is a particle accelerator. It does require, however, complex computers to operate.